For me, running these days, is a form of meditation. Essentially, I am running on automatic. To run faster, I have to think, “Run harder.”
How do we actually run harder? What kind of a neural network helps to run faster?
This post is largely borrowed from a Scientific American blog written by Scicurious and published on July 16, 2012: When you run fast, your brain runs faster. This is most of what she wrote:
“… Today we’re going to talk about a paper that may have worked out a tiny piece of how the brain might deal with things like increased speed. How does your brain keep up with your feet?
By running a little faster.
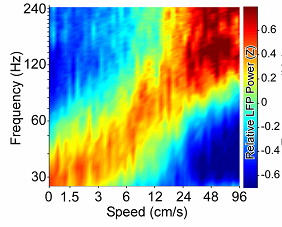
[Above is Figure 2E in Ahmed and Mehta (2012). It is a spectrogram showing the relative local field potential (LFP) at each frequency as a function of both speed and frequency.]
To understand how this works. We need to talk about two major things: place neurons, and oscillatory networks.
Place neurons are just what they sound like. In the hippocampus, certain cells will fire in response to certain places. When you record cell firing from a rat’s brain with an electrode array, you can actually see and hear the neurons firing as a rat looks around a place it has been before (and this is definitely one of the awesome and defining moments of a young neuroscientist), certain neurons will fire for, say, the left corner, and others will fire for the bottom right.
But here’s where we get to oscillation. You see, a neuron doesn’t fire alone. It fires repetitively with large numbers of nearby neurons, in turn triggering other neurons to fire, resulting in a firing network of neurons. These networks fire at certain frequencies and patterns, which we describe by their frequencies, with names like theta and gamma and beta.
The question is, how do things like place cell firing and neural oscillations vary as you move? The authors of this study wanted to see just how place cell firing varied as a function of an animal’s speed. To do this, they hooked electrode arrays into the hippocampi of rats, and watched the oscillations of their place cells as they ran a maze. They set up a nice large Y Maze, with rewards at all the ends, and let the animals run it as fast as they wanted. They looked to see how the activity in the hippocampus varied as a function of speed.
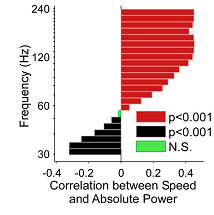
[Above is Figure 4G in Ahmed and Mehta. Ahmed and Mehta (2012). It is a spectrogram showing the relative local field potential (LFP)at each frequency as a function of both speed and frequency.]
What you can see here is a correlation between the power of the gamma oscillations of the place neurons, and the speed of the animals as they ran the maze. It’s not an increase in the frequency of the oscillation, because that would just move it up the [frequency] scale, and it would no longer be a gamma oscillation. Rather, it is an increase in power, more neurons firing in synchrony. They found that as speed increased, there was a strong correlation for the gamma oscillation to increase in power as well. …”
“But what does this mean? What is causing the increase in the gamma oscillations? Well, increased power of gamma oscillations has to be triggered by something. In this case, the authors hypothesized that excitatory input on to interneurons in the hippocampus, which provide inhibitory input to the place cells, might be determining the increase power. When they recorded from interneurons as well as place cells, the authors found that the interneuron firing rate correlated with the increase in gamma power. This suggests that the increased excitatory input stimulated interneurons to increase inhibitory input as the rat runs faster, which might increase synchronicity and might be driving the increased power in the gamma oscillations …”
“But what is the purpose of this increase in gamma oscillations? Since these are taking place in the hippocampus, they may well have something to do with learning and memory. As I mentioned above, neurons in the hippocampus encode things like place, they fire in response to where you are in an area. When they fire synchronously, they do so in the gamma range, resulting in these gamma oscillation. …”
“And this is an important thing to think about. Because, if you’re running FASTER, you have to determine where you are [FASTER] as well! This means that you’ll need to process your spatial information faster. How do you do that? Well, the authors propose two options. First, you could leave out bits of spatial recognition processing, skipping certain elements so you only get the most essential information. But you could also just…run the whole spatial processing sequence faster. And the authors hypothesize that this second option may be the right one. The increased gamma oscillations that correlate with speed are how the animal keeps up with its own feet, processing spatial knowledge faster as it runs faster. The faster transitions might allow the hippocampal cells to encode place no matter how fast you’re going.
But of course, this isn’t the end of the line. This paper shows that increased excitatory input to hippocampal interneurons increases the gamma oscillations, and this varies in speed as you run….but where [do the authors suggest is this] excitatory input itself coming from? From the visual system? The vestibular system? Both? While this provides a cool and interesting piece of the puzzle, there’s always still another step in knowing how we walk and run. And it’s puzzles like that that stop a neuroscientist in their tracks.”
And exercise psychophysiologists, too! Very intriguing that more leg muscle cell power used is associated with more hippocampal place cell power.
Tune back in next week when the topic is Minding the Bend.
Reference
Ahmed OJ, & Mehta MR (2012). Running speed alters the frequency of hippocampal gamma oscillations. The Journal of neuroscience : the official journal of the Society for Neuroscience, 32 (21), 7373-83 PMID: 22623683
About the Scientific American Post Author: Scicurious is a PhD in Physiology, and is currently a postdoc in biomedical research. She loves the brain. And so should you. Follow on Twitter @Scicurious.